What We Review
Introduction
Efficient energy generation is an important priority for many countries that seek to reduce their dependence on nonrenewable energy sources. Although fossil fuels provide much of today’s electricity and transportation power, they also contribute to air pollution and greenhouse gas emissions. Consequently, scientists and policymakers often explore alternative energy solutions that can produce electricity without adding large amounts of carbon dioxide to the atmosphere. One technology that has attracted considerable attention is the hydrogen fuel cells.
Hydrogen fuel cells offer the possibility of a cleaner energy pathway when their hydrogen comes from renewable sources. They operate by combining hydrogen and oxygen, producing electricity and water in the process. Because only water leaves the device, a well-managed hydrogen fuel cell system releases minimal emissions. Therefore, hydrogen fuel cells play a role in ongoing efforts to address environmental issues and transition toward sustainable energy solutions.
What Are Hydrogen Fuel Cells?
A hydrogen fuel cell is an electrochemical device that transforms chemical energy into electrical energy using hydrogen gas as a fuel. Its basic structure typically includes two electrodes (an anode and a cathode) separated by an electrolyte membrane. The design ensures that hydrogen, introduced at the anode, is separated into protons and electrons. Meanwhile, oxygen from the air enters the cathode. The electrons travel through an external circuit, thereby generating electricity, while protons move through the electrolyte to combine with oxygen on the other side.
When discussing the reaction in chemical terms, the overall process can be written as: 2H_2 + O_2 \rightarrow 2H_2O.
This formula shows that modern hydrogen fuel cells are not fundamentally different from a simple chemical reaction. However, the specialized design allows for controlled splitting of hydrogen into charged particles. For instance, when a car runs on a hydrogen fuel cell, hydrogen gas is introduced into the fuel cell stack. The stack’s architecture ensures that chemical energy is converted directly to electrical energy, which can power an electric motor.
Example of a Hydrogen Fuel Cell in Action
Visualize a small chamber with a membrane in the middle. Hydrogen enters on one side, while oxygen enters on the opposite side. The membrane permits protons to pass through but directs electrons to an external circuit. Therefore, once the hydrogen atoms split into protons and electrons, the electrons flow around this external loop, creating an electric current. Eventually, the electrons rejoin the protons and oxygen ions, forming water as the final product.
How Do Hydrogen Fuel Cells Generate Electricity?
Hydrogen fuel cells follow a few fundamental steps to produce electric power. Understanding each step highlights both the efficiency and the complexity of this process.
- Hydrogen Storage
- First, hydrogen must be stored in a tank, often at high pressure. Proper containers are necessary to keep hydrogen in a safe and readily available form. Storing hydrogen efficiently is essential for fuel cells, especially those used in transportation.
- Hydrogen and Oxygen Reaction
- Next, inside the fuel cell, hydrogen and oxygen come into close proximity without mixing freely. This arrangement is crucial because the direct mixture of hydrogen and oxygen can be explosive. Instead, the system channels hydrogen to an anode, where it is split into ions and electrons. Meanwhile, oxygen is supplied to a cathode and gains electrons at the other side of the cell.
- Production of Electricity and Water
- The electrons, forced to move through an external circuit, generate an electrical current that can power devices. Finally, the hydrogen ions and electrons reunite with oxygen to form water. Therefore, water is the primary emission.
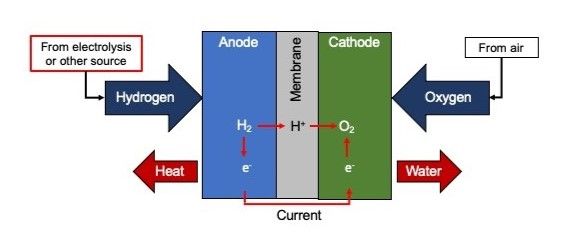
Example: Comparing Hydrogen Oxidation to Baking
Baking requires precise ingredients, heat, and time to produce a finished product. Similarly, hydrogen fuel cells operate by combining the right “ingredients” (hydrogen and oxygen) at carefully regulated conditions. If the procedure is managed correctly, the “dough” of hydrogen and oxygen turns into a finished “loaf” of electricity, leaving water as the only by-product.
Advantages of Hydrogen Fuel Cells
Hydrogen fuel cells present several compelling benefits that could reshape the energy landscape.
- Low Emissions
- When hydrogen is produced from water using renewable energy sources (such as solar or wind), fuel cells release virtually no carbon dioxide. Thus, they can help slow the addition of greenhouse gases to the carbon cycle, which is crucial for managing climate change.
- Renewable Potential
- A hydrogen fuel cell gains greater environmental value if the hydrogen it uses is generated from renewable energy. Energy sources like solar power or wind turbines can facilitate a process called electrolysis, in which water is split into hydrogen and oxygen. Therefore, the overall cycle can be near-zero in net carbon emissions.
- Versatility in Applications
- Hydrogen fuel cells can power vehicles, generate electricity for buildings, and run industrial equipment. They scale from small units for portable devices to large systems for central power plants. Therefore, the technology holds promise in multiple sectors, including transportation, manufacturing, and household energy.
Example: Traditional Fossil Fuels vs. Hydrogen Fuel Cells
A car powered by fossil fuels typically produces carbon monoxide, carbon dioxide, and other harmful gases. On the other hand, a car powered by a hydrogen fuel cell mostly emits water vapor. Therefore, adopting hydrogen-based transportation can significantly reduce smog and greenhouse gas formation in urban areas.
Challenges of Hydrogen Fuel Cells
Although hydrogen fuel cells can offer environmental benefits, several hurdles must be addressed before they become a widespread solution.
- Economic Costs
- Fuel cell systems are often expensive to produce. Specialized materials, including precious metal catalysts such as platinum, raise manufacturing costs. Moreover, storing and transporting hydrogen requires advanced infrastructure. Thus, governments, research institutions, and companies must work together to reduce these expenses.
- Energy Requirements for Hydrogen Production
- If hydrogen comes from electrolysis, it demands a large amount of electricity. Nonrenewable sources still dominate the grid, hence hydrogen production may indirectly cause carbon dioxide emissions. Until more renewable energy capacity is installed, the overall impact of hydrogen could be less beneficial than intended.
- Infrastructure Limitations
- Widespread hydrogen fueling stations do not yet exist in most parts of the world. Consumers need the convenience of easily accessible hydrogen in order to adopt fuel cell vehicles. Furthermore, building this infrastructure from scratch can be costly and time-consuming.
Example: Relating Costs to Everyday Expenses
Consider buying a new smartphone that requires a special charger not sold in regular stores. Initially, transporting and purchasing this unique charger may be more expensive. Similarly, hydrogen technology requires specialized equipment and entire fueling networks, which raise prices for everyday use compared to traditional gasoline.
Hydrogen Production Methods
Producing hydrogen sustainably is crucial for ensuring that fuel cells maintain their low environmental footprint. Two common methods are frequently discussed:
- Electrolysis
- This process uses electric current to split water into hydrogen and oxygen. The reaction can be represented generally as 2H_2O \rightarrow 2H_2 + O_2.
- If the electricity comes from renewable energy, electrolysis yields hydrogen with minimal carbon emissions. However, if fossil fuels are used to power the process, the overall benefit is diminished.
- Steam Methane Reforming
- Often abbreviated as SMR, this method involves reacting natural gas (methane) with steam to produce hydrogen and carbon monoxide. The chemical reaction can be approximated as CH_4 + H_2O \rightarrow CO + 3H_2.
- Although this is presently the most common and cost-effective way to produce hydrogen, it does emit carbon dioxide and thus has a direct impact on the carbon cycle.
Example: Water Splitting Analogy
Splitting water through electrolysis is akin to breaking a Lego model into its component bricks. Initially, the “model” is a water molecule, but with the right amount of energy, it separates into smaller building blocks: hydrogen and oxygen. Carefully reassembling these blocks in a fuel cell later produces electricity and water.
Environmental Impacts
Hydrogen fuel cells can have a positive influence on air quality and climate goals. For instance, a properly managed system using renewably sourced hydrogen generates only water vapor as a by-product. Consequently, no direct carbon dioxide enters the atmosphere.
However, the environmental footprint increases if fossil fuels dominate hydrogen production. Under those conditions, the upstream process might still release carbon dioxide and other pollutants. Furthermore, some industrial methods intensify local water usage, which can strain regional water resources. Therefore, careful planning and policy support are essential to ensure that hydrogen’s ecological advantages outweigh its drawbacks.
Example: Garden Analogy
Imagine watering a garden using rain collected in sustainable barrels versus drawing water from a municipal source during a drought. In both cases, plants can survive, but the environmental impacts differ significantly. In the same way, generating hydrogen with renewable energy is more beneficial for the “garden” (the environment) than relying on nonrenewable inputs.
Future of Hydrogen Fuel Cells
Multiple research institutions are advancing fuel cell technology to make it more efficient and less costly. Several automotive manufacturers have launched hydrogen-powered vehicles, showcasing potential for broader adoption. Moreover, fuel cell buses and trucks are gradually appearing in select cities, indicating that heavy transportation could shift away from diesel.
Policymakers in various countries also recognize the importance of hydrogen in the renewable energy mix. Some governments provide subsidies or incentives to promote hydrogen infrastructure, reducing initial costs for manufacturers and consumers. However, further progress requires continued investment, technological breakthroughs, and global cooperation. In the coming decades, larger fleets of hydrogen vehicles, hydrogen-based power plants, and local refueling networks may become more common if supportive policies remain in place.
Example: Real-World Applications
A housing complex might use rooftop solar panels to generate electricity for electrolyzing water, thereby creating hydrogen on-site. That hydrogen can then power fuel cells to supply electricity and heating when solar generation is insufficient. Such integrated systems illustrate how hydrogen can complement other renewable energies.
Conclusion
Hydrogen fuel cells represent a promising avenue for reducing reliance on nonrenewable energy sources, especially if their hydrogen comes from low-carbon processes such as wind or solar-based electrolysis. Although several challenges remain, including infrastructural needs and production costs, hydrogen fuel cell innovations continue to evolve. In many parts of the world, engineers and policymakers are working together to shape a future where hydrogen plays a significant role in clean energy applications.
If developed responsibly, hydrogen fuel cells can drastically cut emissions compared to typical fossil fuels, reduce the strain on the carbon cycle, and open new possibilities for sustainable energy solutions. Therefore, continued research and policy support may accelerate the adoption of hydrogen fuel cell technologies, making them an integral part of a more environmentally conscious future.
Key Vocabulary
- Nonrenewable Energy: Energy sourced from finite resources, such as coal or petroleum, that cannot be replenished quickly.
- Electrolysis: A process using electricity to split water into hydrogen and oxygen gas.
- Emission: Substances released into the environment as a by-product of a process; in hydrogen fuel cells, the main emission is water.
- Steam Methane Reforming: A common method of producing hydrogen from natural gas, involving a reaction with high-temperature steam.
- Carbon Cycle: The movement of carbon between the atmosphere, land, and oceans, influenced by both natural processes and human activities.
- Infrastructure: The necessary physical and organizational structures, such as fueling stations and pipelines, that support a technology’s use.
Sharpen Your Skills for AP® Environmental Science
Are you preparing for the AP® Environmental Science test? We’ve got you covered! Try our review articles designed to help you confidently tackle real-world AP® Environmental Science problems. You’ll find everything you need to succeed, from quick tips to detailed strategies. Start exploring now!
- AP® Environmental Science: 6.8 Review
- AP® Environmental Science: 6.9 Review
- AP® Environmental Science: 6.10 Review
Need help preparing for your AP® Environmental Science exam?
Albert has hundreds of AP® Environmental Science practice questions, free response, and full-length practice tests to try out.