What We Review
Introduction
Nuclear power is an important energy source in today’s world. It relies on using the heat released by splitting atomic nuclei, primarily Uranium-235, to produce electricity. This energy generation method plays a vital role in discussions about sustainable power, the uses of nuclear power, and climate change because it produces much lower air‑polluting emissions than fossil fuels. However, it also presents unique challenges, such as radioactive waste management. To understand nuclear power, we explore how it works, the reasons we use it, and the potential risks involved.
Nuclear power is central to AP® Environmental Science because it illustrates the complex balance between meeting human energy demands and protecting environmental health. As population growth models continue to show rising energy needs, scientists and policymakers frequently examine nuclear power’s pros and cons. The following sections discuss how nuclear fission occurs, the process of generating electricity, the uses of nuclear power, and the environmental impacts of using this technology.
What Is Nuclear Power?
Nuclear power is generated through a process called fission, in which the nucleus of a heavy atom splits into smaller parts. Large atoms, such as Uranium-235, are especially useful because the fission of these atoms releases significant energy. This energy appears primarily as heat and radiation.
Explanation of Nuclear Fission
Nuclear fission occurs when a neutron strikes an atom’s nucleus, causing the nucleus to split. This splitting process generates smaller atoms (known as fission products), additional neutrons, and a substantial amount of energy. The energy released can be compared to splitting a cookie: one cookie is broken into smaller pieces, but unlike a simple cookie crumbling, the additional neutrons from Uranium-235 can spark a chain reaction, continuing the fission process. Nuclear power plants carefully control the chain reaction to ensure the energy release is steady rather than explosive.
Uranium-235 is well suited for fission because it releases more neutrons on average, which can facilitate a consistent reaction. Despite its benefits, Uranium-235 remains radioactive for a very long time, creating concerns about safe disposal and potential contamination. This issue will be examined later when discussing nuclear waste.
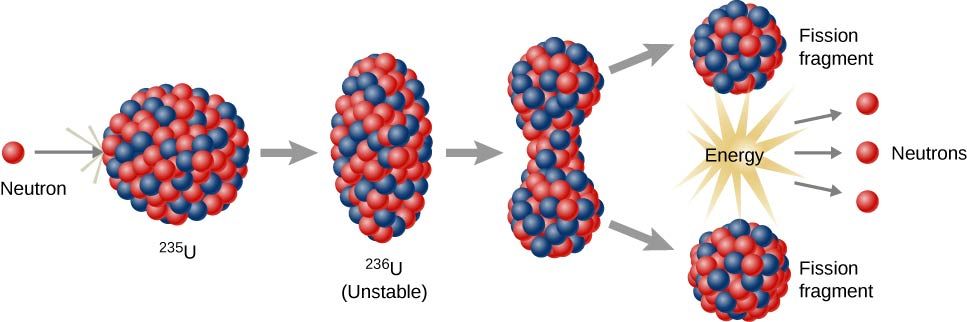
How Nuclear Power Is Generated
Producing electricity from nuclear fission involves a relatively straightforward series of steps. Each step, however, requires stringent safety measures and carefully designed equipment.
- Fuel rods containing Uranium-235 are placed in a nuclear reactor.
- A neutron starts the chain reaction by striking Uranium-235 atoms in these rods.
- Fission of Uranium-235 releases heat, which warms a surrounding fluid (often water).
- The superheated fluid turns to steam, which drives a turbine.
- The turbine is connected to a generator that produces electricity.
Simple Analogy: Making Tea
A helpful analogy involves boiling water to make tea. A heating element warms the kettle, transforming water into steam. In a nuclear power plant, fission is the heating element, steam is produced as water boils, and the steam’s pressure spins a turbine. Finally, the turbine’s motion generates electricity that can be sent through power lines.
Just as leaving a kettle unattended can create hazards like overheating, improper control of nuclear fission poses substantial risks. Therefore, nuclear reactors rely on safety mechanisms, including control rods that regulate or halt the chain reaction by absorbing some of the neutrons.
The Uses of Nuclear Power
Nuclear power is primarily employed for electricity generation, which supplies households, schools, hospitals, and industries with a stable energy source. However, additional uses exist:
- Medical applications, such as the production of radioactive isotopes used in diagnostic imaging and cancer treatments.
- Industrial processes that require high levels of heat or radioactive tracing.
- Naval propulsion, where nuclear reactors power submarines and aircraft carriers.
One key point is that nuclear power does not directly emit carbon dioxide, making it an attractive low‑carbon option in conversations about the carbon cycle and how to reduce greenhouse gas emissions. Although it is not a renewable energy source—Uranium-235 is a nonrenewable material—it is often compared to renewable energy technologies because of its potential to lessen air pollution.
Environmental Impact of Nuclear Power
Nuclear power’s positive and negative environmental impacts necessitate careful analysis.
Benefits
Many environmental scientists classify nuclear power as a cleaner energy source compared to typical fossil fuel–based electricity generation. It produces fewer air pollutants such as carbon dioxide, sulfur dioxide, and particulate matter. Therefore, substituting some fossil fuel plants with nuclear plants may reduce the impact on global warming and associated climate change problems.
Drawbacks
However, nuclear energy comes with significant challenges:
- Thermal Pollution: Nuclear reactors often use rivers, lakes, or coastal waters for cooling, returning heated water to these sources. This warmed water can harm aquatic ecosystems, especially organisms sensitive to temperature changes.
- Hazardous Solid Waste: After the fission reactions occur, spent fuel rods remain highly radioactive. These rods must be stored in secure facilities, often underground. Failure to handle this waste carefully creates risks for organisms and ecosystems.
- Nonrenewable Resource: Uranium-235 is finite within Earth’s crust. Even though the chain reactions release large amounts of energy, the supply of Uranium‑235 is limited.
Therefore, nuclear facilities invest heavily in waste management research. Suggestions include deep geological storage sites to shield living organisms from radiation and ongoing work to minimize waste volume. Effective policies address both current and future storage challenges, ensuring nuclear energy remains as safe as possible.
Accidents and Their Lessons
Nuclear accidents draw attention to potential dangers when controlling radioactive materials:
- Three Mile Island (1979): A partial reactor meltdown in Pennsylvania. The event led to reforms that improved safety protocols and communication about nuclear incidents.
- Chernobyl (1986): A reactor explosion in what is now Ukraine caused widespread contamination across Europe. This accident prompted a global review of nuclear plant design and radiation containment.
- Fukushima (2011): Following a tsunami, reactor cores at the Fukushima Daiichi plant overheated and released radiation. Governments worldwide re‑examined coastal facility designs, emergency power systems, and disaster preparedness plans.
These cases underscore the need for strict safety standards, effective containment structures, and robust backup systems. Short‑term consequences include local radiation exposure, environmental contamination, and health risks for nearby populations. Long‑term outcomes involve the persistence of radioactive materials and ecological damage, challenging researchers and policymakers to mitigate harm.
Understanding Radioactivity
Radioactivity occurs when unstable atomic nuclei release energy in the form of radiation. This process modifies the nucleus, sometimes turning one element into another. Radioactivity is central to nuclear power generation, where controlled reactions release consistent energy. It is also central to waste management and accident response, as materials remain radioactive for extended periods.
Half-Life and Its Significance
The concept of half-life is vital in understanding radioactive materials. A half-life is the time required for half the atoms in a radioactive sample to decay. For instance, if a 100‑gram sample of Uranium-235 has a certain half-life, it will become 50 grams of Uranium‑235 (plus decay products) after one half-life period.
Mathematically, the amount of radioactive material over time can be calculated using:
N(t) = N_0 \left(\frac{1}{2}\right)^{\frac{t}{t_{1/2}}}Here,
- N_0 is the initial quantity of the radioactive substance.
- N(t) is the quantity remaining after time t.
- t_{1/2} is the half-life of that radioactive substance.
If Uranium-235 is disposed of after use in a reactor, it remains hazardous for tens of thousands of years because of its long half-life. This poses storage and containment demands, ensuring that no significant leaks of radioactive materials seep into the surrounding environment.
Conclusion
Nuclear power’s value lies in providing large amounts of relatively clean electricity, making it a key component in the uses of nuclear power. At the same time, it carries risks related to waste disposal, reactor safety, and thermal pollution. Understanding nuclear fission, radioactivity, and half-life is essential for evaluating how nuclear power fits into energy policies. Careful management, along with learning from incidents like Three Mile Island, Chernobyl, and Fukushima, serves to improve safety protocols and safeguard ecosystems.
Continuing exploration of nuclear technology can advance the design of reactors that minimize waste and reduce the chance of serious accidents. Responsible use demands sound policy, rigorous research, and ongoing public engagement. Deeper study of nuclear power not only enhances knowledge about environmental systems but also guides balanced decisions that address growing energy demands while reducing the negative impacts on the planet.
Key Vocabulary
- Fission: The process by which a heavy atomic nucleus splits into smaller parts, releasing energy and additional neutrons.
- Uranium‑235: A naturally occurring, fissile isotope of uranium commonly used as fuel in nuclear reactors.
- Radioactivity: The spontaneous emission of radiation from unstable atomic nuclei.
- Half‑life: The time required for half of the radioactive atoms in a sample to undergo decay.
- Thermal Pollution: The release of heated water or air into the environment, altering local temperature conditions and potentially harming ecosystems.
Sharpen Your Skills for AP® Environmental Science
Are you preparing for the AP® Environmental Science test? We’ve got you covered! Try our review articles designed to help you confidently tackle real-world AP® Environmental Science problems. You’ll find everything you need to succeed, from quick tips to detailed strategies. Start exploring now!
Need help preparing for your AP® Environmental Science exam?
Albert has hundreds of AP® Environmental Science practice questions, free response, and full-length practice tests to try out.