What We Review
Introduction
Solar radiation drives many fundamental processes on Earth, influencing climate, weather, and life itself. It is also a core topic in AP® Environmental Science. The following sections explain how solar radiation and Earth’s tilt create our seasons, why latitudes receive different amounts of sunlight, and why day lengths vary throughout the year. By examining these patterns, it becomes easier to see how energy from the sun underpins much of our planet’s environmental dynamics.
Solar radiation is the energy emitted by the sun, traveling across space in the form of electromagnetic waves. When these waves reach Earth, they power photosynthesis, warm the planet, and set the stage for complex interactions between the biosphere, atmosphere, and hydrosphere. Understanding how solar radiation works—and how Earth’s tilt, latitude, and seasons interrelate—enhances comprehension of broader environmental topics, such as the carbon cycle and the formation of distinct ecosystems.
What Is Solar Radiation?
Solar radiation encompasses all electromagnetic energy arriving from the sun. This energy stream is referred to as \text{insolation}, or “incoming solar radiation.” Insolation is Earth’s main energy source and is crucial for understanding environmental phenomena like cloud formation, ocean currents, and even the population growth model of certain organisms (because solar radiation influences primary productivity).
- Solar radiation includes various wavelengths, such as visible light and ultraviolet rays.
- Because Earth is round, not every point receives the same intensity of this sunlight.
Example: Visualizing Solar Radiation
A simple demonstration uses a lamp as the sun and a small globe as Earth:
- Place the lamp at one side of a dark room.
- Shine the lamp on the globe.
- Note how the side facing the lamp is illuminated while the opposite side remains dark.
- Observe that the globe’s surface closer to the center of the light beam receives more direct radiation, creating a brighter spot. Areas near the edges get more diffused light.
This exercise shows how insolation depends on the angle at which sun rays strike Earth’s surface.
The Earth’s Tilt and Its Impact on Seasons
One key reason for the Earth’s seasons is its axis of rotation. Earth’s axis is tilted at an angle of approximately 23.5^\circ with respect to the plane of its orbit around the sun. This tilt ensures that different latitudes receive varying amounts of solar radiation throughout the year.
- When the Northern Hemisphere tilts toward the sun, it experiences summer, as it receives more direct sunlight.
- Meanwhile, the Southern Hemisphere—tilting slightly away—experiences winter at that same time.
Example: A Year-Long Journey
Consider a model or animated video that tracks Earth in its orbit:
- Set the globe at different positions around a central light source representing the sun.
- Mark the tilt to remain constant, always pointing to the same direction in space (imagine a fixed star in the background).
- In June, the Northern Hemisphere leans toward the sun, receiving more insolation and creating the longest summer days.
- Six months later, Earth’s position changes. Now, the Southern Hemisphere receives more direct rays, leaving the Northern Hemisphere in winter.
Through this year-long orbit, the consistent tilt explains why, at different times, various regions enjoy longer or shorter days.
The Angle of the Sun’s Rays
The angle at which sunlight hits Earth matters greatly. When the sun’s rays strike at or near a right angle, solar radiation is concentrated over a smaller surface area. Thus, these rays deliver more energy per unit area. In contrast, when the rays arrive at a slant, the same energy spreads out over a larger area, weakening the intensity.
- Near the equator, sunlight arrives closer to a right angle, boosting the amount of solar energy received.
- Closer to the poles, the angle is more oblique, resulting in reduced solar intensity.
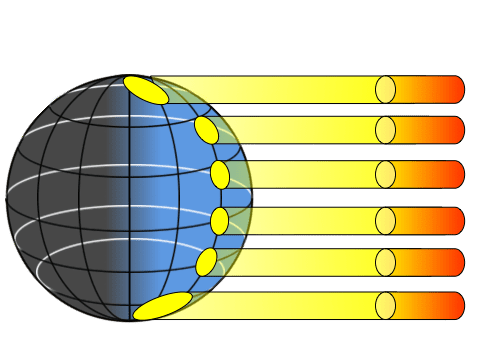
Example: Direct vs. Angled Sunlight
On a sunny day, observe the difference between standing directly under the sun at noon (when the sun is overhead) versus standing in the late afternoon when the sun is near the horizon. At noon, the sunlight feels more intense because it covers a smaller area on the ground. By evening, the sun’s rays skim the surface at a shallow angle, delivering weaker warmth.
Mathematically, the intensity I of sunlight can be approximated if one knows the angle of incidence \theta by using I = I_0 \times \cos(\theta), where I_0 is maximum intensity when the rays are perpendicular. Notice that as \theta increases (rays become more slanted), the value of \cos(\theta) decreases, reducing I.
Seasonal Variation in Solar Radiation
Seasonal variation in solar radiation depends on where the sun’s most direct rays strike Earth and how long the sun is above the horizon each day. During summer months in the Northern Hemisphere, days are extended, and sunlight arrives more directly. Therefore, total daily insolation is much higher compared to winter days, when sunlight is indirect and daylight hours are fewer.
- On the longest summer day in June for the Northern Hemisphere, locations at higher latitudes can experience very long days (in places like Alaska, the sun may not set at all).
- On the shortest winter day in December, the same locations might see only a few hours of daylight, reducing total insolation.
Example: Summer vs. Winter Insolation
- Take a location at 45^\circ,\text{N}.
- In summer, that location receives sunlight for roughly 15 to 16 hours per day, with the sun higher in the sky at noon.
- In winter, it might receive only 8 to 9 hours of daylight, and the sun remains low on the horizon.
- Tracking the temperature variations, one can see how higher levels of solar radiation correlate with warmer summer conditions.
This pattern is part of Earth’s climate regulation, contributing to differences in vegetation, wildlife activity, and even humidity levels in various regions.
The Impact of Latitude on Solar Radiation
Latitude plays a leading role in determining how much solar radiation reaches a given area. Recall that the highest solar radiation per unit area occurs at the equator. Moving toward the poles, the intensity steadily decreases. This geographic gradient helps explain why tropical rainforests exist near the equator, while colder climates dominate higher latitudes.
- Near the equator, high insolation drives temperatures that often favor lush ecosystems, abundant rainfall, and robust plant growth.
- Toward the poles, lower insolation leads to colder climates, permafrost, or ice caps.
Example: Tropics vs. Polar Regions
Analyzing temperature patterns:
- Observe average yearly temperature in a tropical city near 10^\circ,\text{N}. This region typically has little seasonal temperature change, thanks to steady, high-angle sunlight.
- Compare that to a polar region near 70^\circ,\text{N}, which experiences stark contrasts between summer and winter. Summer might have continuous daylight yet cooler temperatures overall, while winter is long and cold with minimal sunlight.
- Because the sun strikes more variably at higher latitudes, seasons become more pronounced, affecting local ecosystems and biodiversity.
Key Vocabulary
- Solar Radiation: Energy from the sun in the form of electromagnetic waves that heats Earth’s surface and atmosphere.
- Insolation: Incoming solar radiation (measured at Earth’s surface) that varies with latitude and season.
- Tilt of Earth’s Axis of Rotation: The approximately 23.5^\circ inclination that causes varying sunlight angles and day lengths over the year.
- Latitude: A geographical coordinate that determines how directly sun rays strike a location; crucial for climate patterns.
- Angle of the Sun’s Rays: The angle at which sunlight reaches Earth’s surface, affecting the intensity of solar radiation.
Conclusion
Seasonal changes in solar radiation occur due to Earth’s tilt, the angle of incoming sunlight, and latitude. Together, these factors help shape the planet’s diverse climates, ranging from tropical forests at the equator to icy polar conditions near the poles. A solid understanding of these dynamics is pivotal in AP® Environmental Science because they connect to broader environmental processes and influence how humans manage resources, from renewable energy to agricultural planning.
Sharpen Your Skills for AP® Environmental Science
Are you preparing for the AP® Environmental Science test? We’ve got you covered! Try our review articles designed to help you confidently tackle real-world AP® Environmental Science problems. You’ll find everything you need to succeed, from quick tips to detailed strategies. Start exploring now!
- AP® Environmental Science: 4.3
- AP® Environmental Science: 4.4
- AP® Environmental Science: 4.5
- AP® Environmental Science: 4.6
Need help preparing for your AP® Environmental Science exam?
Albert has hundreds of AP® Environmental Science practice questions, free response, and full-length practice tests to try out.